- 1Department of Pharmacology and Therapeutics, Faculty of Veterinary Medicine, Damanhour University, Damanhour, Egypt
- 2Department of Pharmaceutical Chemistry, College of Pharmacy, Taif University, Taif, Saudi Arabia
- 3Department of Food Hygiene, Faculty of Veterinary Medicine, Benha University, Benha, Egypt
- 4Department of Bacteriology, Immunology, and Mycology, Faculty of Veterinary Medicine, Suez Canal University, Ismailia, Egypt
- 5Department of Biochemistry and Molecular Biology, Faculty of Science, Egerton University, Njoro, Kenya
- 6Department of Pharmacy, University of Rajshahi, Rajshahi, Bangladesh
- 7Department of Pharmacognosy, Faculty of Pharmaceutical Sciences, Government College University, Faisalabad, Pakistan
- 8Department of Pharmacy, Atish Dipankar University of Science and Technology, Dhaka, Bangladesh
- 9Pratyasha Health Biomedical Research Center, Dhaka, Bangladesh
- 10Department of Natural Sciences, Faculty of Pure and Applied Sciences, Precious Cornerstone University, Ibadan, Nigeria
- 11Department of Medical Microbiology and Immunology, Faculty of Medicine, Assiut University, Asyut, Egypt
- 12Faculty of Medicine, University of Porto, Porto, Portugal
- 13Institute for Research and Innovation in Health (i3S), University of Porto, Porto, Portugal
- 14Laboratory of Neuropsychophysiology, Faculty of Psychology and Education Sciences, University of Porto, Porto, Portugal
- 15Department of Natural Products Research, Dr. Koirala Research Institute for Biotechnology and Biodiversity, Kathmandu, Nepal
- 16Laboratory of Biotechnology, Faculty of Science and Technology, University of Macau, Taipa, Macau
- 17Microbiology Division, Department of Botany, Gauhati University, Guwahati, India
- 18Departamento de Ciencias del Ambiente, Facultad de Química y Biología, Universidad de Santiago de Chile, Santiago, Chile
- 19Biological Sciences Department, Federal University of Lavras (UFLA), Lavras, Brazil
- 20Université Aix-Marseille, Institut de Neuro-Physiopathologie (INP), UMR 7051, Faculté de Pharmacie, Marseille, France
Coronavirus disease (COVID-19) is a global health challenge, caused by the severe acute respiratory syndrome coronavirus-2 (SARS-CoV-2) triggers a plethora of respiratory disturbances and even multiple organs failure that can be fatal. Nutritional intervention is one of the key components toward to a proper management of COVID-19 patients, especially in those requiring medication, and should thus be considered the first-line treatment. Immuno-modulation and -stimulation are currently being explored in COVID-19 management and are gaining interest by food and pharmaceutical industries. Various dietary combinations, bioactive components, nutrients and fortified foods have been reported to modulate inflammation during disease progression. Dietary combinations of dairy-derived products and eggs are gaining an increasing attention given the huge immunomodulatory and anti-inflammatory properties attributed to some of their chemical constituents. Eggs are complex dietary components containing many essential nutrients and bioactive compounds as well as a high-quality proteins. Similarly, yogurts can replenish beneficial bacteria and contains macronutrients capable of stimulating immunity by enhancing cell immunity, reducing oxidative stress, neutralizing inflammation and regulating the intestinal barriers and gut microbiome. Thus, this review highlights the impact of nutritional intervention on COVID-19 management, focusing on the immunomodulatory and inflammatory effects of immune-enhancing nutrients.
Introduction
Coronavirus disease (COVID-19) triggered by the severe acute respiratory syndrome-coronavirus-2 (SARS-CoV-2) led to multiple respiratory disturbances, multiple organs failure and even death, ultimately representing a global health challenge. The virus, first reported in December 2019, in the Wuhan city – China (1), has spread worldwide and has been classified by the World Health Organization (WHO) as a global pandemic (2, 3). Clinical presentations by most COVID-19 patients requiring admission include severe inflammation, respiratory failure and reduced appetite (4).
Nutritional intervention, also regarded as the first-line treatment, is among the core components involved in comprehensive management of COVID-19 patients under medication. Available clinical evidence reveals that although people of all ages are susceptible to infection, a poor prognosis and higher mortality rate has been reported in malnourished elderly individuals, immunocompromised patients and even in those with chronic diseases (5). In this sense, not only does good nutrition enhance body immunity against diseases, including COVID-19, but also shortens the recovery period (6). The elderly are especially susceptible to infection, owing to their body’s declining physiological and immunological efficiency. This increases the likelihood of infections, being severe and refractory, thereby presenting an immunity challenge to the elderly who happens to contract an infectious pathogen. This elucidates the importance of systematic nutritional derangements in COVID-19 patients since immunity is weakened by inadequate nutrition (7, 8). Currently, immune modulation and stimulation is being increasingly explored for COVID-19 management and has gained interest by both food and pharmaceutical industries. In fact, the immune response is crucial for maintaining healthy human physiology by detecting and eliminating pathogens, aging, or cancer cells (9). Briefly, immunomodulation refers to the immune system ability to regulate fatal illnesses, such as acquired immunodeficiency syndrome caused by human immunodeficiency virus (HIV) (10, 11). Currently used therapeutic immunomodulators include penicillamine, cyclosporine A, cyclophosphamide, pidotimod, levamisole, thiocarbamate, imiquimod, prostaglandin, tilorone, and niridazole (12–16). However, these drugs are usually associated with undesired side effects. In addition, since no effective drug is currently available for COVID-19 treatment or adequate early phase vaccine supply for the entire populace, nutritional enhancement of immune system is the best immediate intervention for preventing SARS-CoV-2 infection. In this sense, this review presents different food sources and nutritional supplements that enhance immune function, such as egg derived proteins, milk and fermented dairy products containing different probiotics (Figure 1), that have been shown to play a key role in preventing COVID-19 and in the management of hospitalized patients with mild clinical presentations.
The primary host’s defense from pathogens and harmful toxins emanates from the immune system (17). Two categories of the immune system include the adaptive and innate subsystems. The innate immune subsystem also referred to as native/natural immunity is non-specific and forms the layer of protection, usually through mechanical barriers of entry, such as the skin and mucosal tissues (17). Other forms of native immunity involve the non-specific inflammatory components, such as interferons, defensins, and cytokines, and also incorporate the bone marrow components, such as the basophils, monocytes, dendritic cells, macrophages, eosinophils, and neutrophils. As critical first-line of defense, neutrophils and macrophages are also of pivotal important in phagocytosis, the mechanisms through which macrophages recognize and neutralize pathogens and defective/cancer cells (18). Macrophages act also as immune regulators by producing different cytokines (i.e., interleukins, interferon gamma, tumor necrosis factors-α) (19). On the other side, an immunomodulator can be stratified as immunosuppressant, immunostimulant, or immunoadjuvant. In this perspective, immunotherapy can be defined as the host’s immunity modulation with the desired outcome of disease treatment or management (20).
In contrast to innate immunity, the specific response by the immune system is referred to as adaptive/acquired/specific. This kind of immune response is usually the second-line of defense and involves B and T lymphocytes (20). Adaptive immunity can either be cell-mediated or humoral immune response, where humoral immunity is mediated by B-lymphocytes through antibodies production, that are specifically directed toward the pathogen, whereas cell-mediated immune responses are initiated by T lymphocytes complexing with infected cells or pathogens, which leads to lysis of the “unwanted” cells and cytokines release, whose primary role is immune regulation. T lymphocytes/cells fall into three subsets, termed as helper (TH), cytotoxic, and suppressor or regulatory T cells. The expression of cluster of differentiation (CD) 8+ cell surface receptors characterizes the cytotoxic lymphocytes. The CD8 surface receptors play a role in recognition of endogenous antigens presented by the major histocompatibility complex class I (MHC-I) from virus-infected cells that have been lysed (Figure 2). In contrast, helper T cells, express CD4+surface receptors that recognize exogenous antigens presented by MHC-II. The reaction mechanism of TH cells entails T and B lymphocytes activation by secreting cytokines that also activate other immune cells (21, 22), whereas the suppressor T cells regulate the immune response by smothering autoimmunity, thereby maintain the self-tolerance.
It has been reported that various dietary combinations, bioactive components, nutrients, and fortified foods modulate inflammation during disease progression (23–25). Eggs represent a complex and controversial dietary component (26) that contains numerous essential nutrients and bioactive compounds, besides being high-quality protein source leading to divergent opinions in dietary recommendation across populations (27, 28).
Egg Structure
Structurally, eggs are composed of albumin (63%), eggshell (9.5%), and yolk (27.5%) (29). Biochemically, they comprise of 75% water, 12% proteins, 12% lipids, various minerals and carbohydrates (30, 31). Though proteins are distributed across the different egg parts, they are mainly contained in the yolk and egg white, while small proportions occur in the eggshell and shell membrane (32, 33). Lipids exclusively occur in the egg yolk mainly as lipoproteins (30, 32), while the bulk of minerals is found in the eggshell. As minor egg components, carbohydrates are found throughout the egg either as free carbohydrates or glycoconjugates (32). The bulk component of egg is the albumen or egg white that constitutes 60% of cumulative egg weight, whereas protein and water add up to other major components (31, 32). The major egg white’s proteins are ovalbumin, ovotransferrin, and ovomucoid. Other proteins include ovomacroglobulin (ovostatin), cystatin, lysozyme, avidin, ovoinhibitor andovomucin that gives the albumen its characteristic viscosity (32).
Dietary Benefits of Eggs
Eggs are rich in complete proteins that promote muscle protein synthesis and maintenance of skeletal mass (34, 35). On average, one large egg provides ~6.3 g protein that is rich in essential amino acids (36). Eggs are affordable nutritious dietary components with significant health benefits (37). These nutrients include vitamins, essential proteins, minerals, fats, and various bioactive compounds. Eggs contain high nutrients to energy density ratio per egg, while also providing numerous essential nutrients (38). For instance, a typical boiled egg weighing ~50 g can provide as much as 78 kcal energy, 0.56 g carbohydrate, 6.29 g protein, and 5.3 g total fat. The total fats from an egg contains up to 1.6, 0.7, 2.0, and 186 mg of saturated, polyunsaturated, monounsaturated fats, and cholesterol, respectively. The micronutrients from eggs include iron, calcium, phosphorus, zinc, potassium magnesium, sodium, and most vitamins except vitamin C. These vitamins include riboflavin, niacin, thiamin, folate, and vitamins A, B6, B12, D, E, and K. Besides providing high quality proteins comparable to breast milk, eggs are also a source of antioxidants. Notable antioxidants from eggs include phosvitin rich in phosphoserines, ovotransferrin that chelates Fe3+and ovalbumin that improves the polysaccharide’s antioxidant activity through covalent binding (39). These antioxidants act by chelating metal ions and scavenging free radicals thereby inhibit the lipids oxidation. Therefore, eggs are potential sources of natural antioxidants that can be used in both cosmetic and food industries. The eggs’ antioxidant activity can ameliorate many degenerative conditions, such as cardiovascular diseases (CVD) in humans (40). However, eggs are not only rich in bioactive components and vital nutrients but also in complete proteins and nutritional cholesterol (28, 41). This controversy has resulted in divergent opinions in dietary recommendation of across populations. Nonetheless, owing to their nutritive quality, eggs, and their derivatives are known to regulate inflammation and modulate immunity.
Eggwhite Proteins: Evidence of Immune System Enhancement
Egg white part is rich in bioactive compounds with antimicrobial activity (42, 43), besides immunoprotective proteins, which include ovalbumin, ovotransferrin, ovomucin, lysozyme, and avidin that account for 54, 12, 3.5, 3.4, and 0.5% of the egg white proteins by weight, respectively (42). These proteins exert antimicrobial and immunomodulatory effect through direct action on inflammatory pathways (44, 45).
Inflammation is a conventional physiological reaction to infection by pathogens and physical tissue disruption, but it has also been associated with chronic metabolic disorders (23, 46). Inflammation can be regulated by both nutritional and bioactive supplements thereby reducing the disease risk or pathogenesis (24, 25).
Eggs are the best example of dietary components with immunomodulatory effects (26). Studies have shown that egg proteins and its derivative peptides act on various immunomodulatory pathways (Table 1). For instance, ovalbumin modified by methylglyoxal stimulates RAW 264.7 macrophages to secrete TNF-α (56). Elsewhere, Rupa et al. (47) reported that heat denatured ovalbumin modulates cytokines production by CD4+ T cells. Heat-denatured ovalbumin also modulates interleukins’ (IL) production by downregulating IL-4 and upregulating IL-10, 12, and 17. Ovalbumin-derived peptides corresponding to amino acids in position 77–84 and 126–134 also enhances the phagocytic activity of macrophages (57) and are reported to enhance immunotherapy (50, 53, 58). Ovotransferrin, another egg white protein, has been shown to stimulate the production of IL-6 and metalloproteinase (MMP) in HD 11 chicken macrophages (59). Xie et al. (59) also reported that ovotransferrin stimulates murine macrophages to produce proinflammatory cytokines through the MAPK signaling pathway. Notably, IL-6 is one of the adaptive immunity components. During chronic inflammation, IL-6 activates T cells, enhances the B cells proliferation and upregulates antibody production. The peptides produced when ovomucin is cleaved by alcalase also express anti-inflammatory activity by inhibiting TNF-mediated NF-κB pathway (49). Tanizaki et al. (60) reported that ovomucin glycoproteins can stimulate macrophages through elevated production of IL-1 and hydrogen. Furthermore, egg white’s cystatin enhances the nitric oxide (NO) production by IFN-γ-activated macrophages as a result of IL-10 and TNF-α cytokines activity (61). Cystatin also affects gingival fibroblasts’ production of IL-6 and 8 cytokines (62). In another study, Sugahara et al. (63) reported lysozyme-mediated production of immunoglobins. Lysozyme-mediated upregulation of IgM synthesis has been shown to be effective in the management of chronic sinusitis bronchitis and sinusitis (55, 64). Elsewhere, Ha et al. (54) reported the immunomodulatory activity of Maillard-type lysozyme-galactomannan conjugate through NO-enhanced cytokines production in macrophages. This immunomodulatory effect also originates from stimulation of ERK, NF-κB, and JNK pathways. Livetin from the egg yolk has been reported to suppress proinflammatory cytokines, such as IL-1β, 6, and 10 and TNF-α in macrophages thereby inhibiting inflammation (52). Immunoglobulins (G1, G2, M, and A) have been shown to possess immunomodulatory properties in the management of bacterial and viral diseases. Yolkin from egg yolk has also been reported to inhibit free radical generation, thereby inhibiting oxidative stress and pro-inflammatory cytokines, like IL-1β, 6, and 10 and TNF-α in macrophages (55), although at high temperatures, the rate of immunoglobulins aggregation is greatly enhanced thereby locking immunoglobulins in aggregates before complete denaturation.
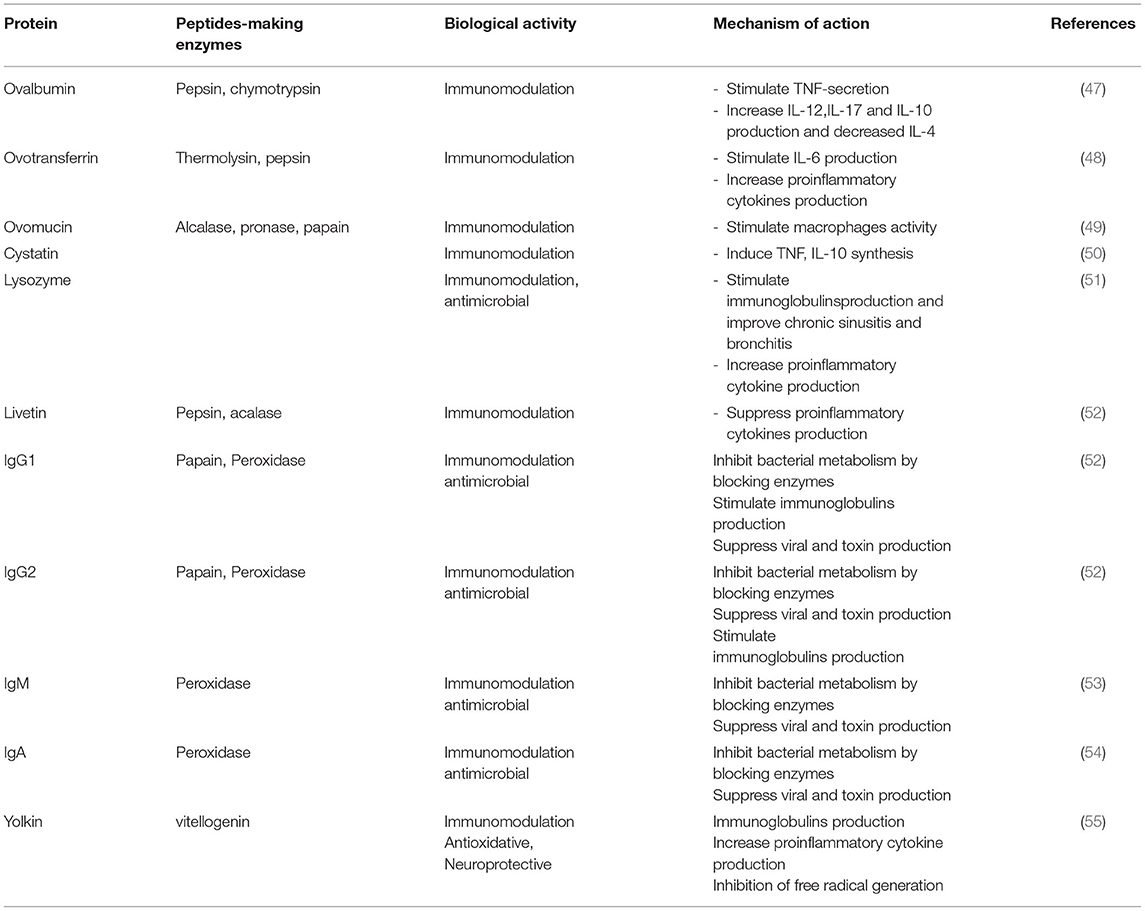
Fermented Dairy Products as Immune-Enhancing Food
Historically, fermentation has been widely used in food/beverage processing and preservation. Food fermentation improves palatability besides enhancing nutrients’ bioavailability. In addition, microbial fermentation can eradicate toxic milk metabolites, such as galactose and lactose, thereby precluding lactose intolerance and galactose accrual (65). This nutritional and health significance results from modulating intestinal microbiome, ultimately enhancing life expectancy. Fermented dairy products have so far been popular in nutritional enhancement and health promotion. For instance, yogurt resulting from synergistic fermentation of lactose in coagulated milk into lactic acid by Streptococcus thermophilus and Lactobacillus bulgaricus action, led to the production of a plethora of bioactive compounds in fermented milk, while others have not yet been characterized. As a result of fermented dairy foods consumption, live commensal microbiota can enhance the gut’s immunological activity and modulate tolerance from foods-derived antigens (66, 67). The synergy between the immune system and gut microbiome is partially understood compared to the bacteria induced by fermentation by-products.
Nutritional Value of Probiotic Yogurt
First, it is essential to highlight that a probiotic yogurt is not only a rich source of proteins and fats but also contains numerous essential nutrients. As much as 8 g fats and 9 g of proteins can be sourced per yogurt serving which is more than enough of the recommended daily protein intake. Yogurt is also rich in micronutrients, such as phosphorus, zinc, calcium (33% recommended daily intake, 1,000 mg), vitamin A (10% recommended daily intake), vitamin B12 (>40% recommended daily intake), and pantothenic acid and riboflavin as per Canada’s health food standards. As a fermented dairy product, yogurt is also potentially effective in alleviating gastrointestinal (GI) complications, such as constipation, lactose intolerance, Helicobacter pylori infection and inflammatory bowel diseases (IBDs) (68, 69). Studies have also suggested that human immunity can be enhanced by yogurt since it influences the intestinal microbiota’s equilibrium thereby stimulating the GI immunity through lactic acid and other bacterial metabolites (70, 71).
Despite this marvelous nutritional significance, a reduced consumption of fermented dairy products is associated with a weakened immunity and elevated risk of infectious diseases. In the COVID-19 perspective, GI complications have been commonly reported in patients whose clinical conditions deteriorates rapidly (72). However, administration of gastric medications need to be approached cautiously on case-by-case basis considering their potential to disrupt the immune response thus interfering with COVID-19 treatments currently under evaluation (73, 74). However, the suggested probiotics’ health effects from fermented dietary products can be realized only when cell viability is reliable (75). So, to ensure probiotics survival through both GI tract and food processing/storage, the minimal recommended presentation of bacteria is 106-107 colony-forming units (CFUs)/mL organism in each food product (76), despite other factors should also be considered when adding probiotic bacteria in fermented food products to ensure the bacteria’s survival. Among them, the compatibility of fermented dairy foods with a wide range of probiotics needs their consideration as “the most efficient delivery vehicle” for probiotics (77, 78), while supplementing fermented milk with probiotics represents an exemplary mode of replenishing the gut microbiota (79).
The Role of Probiotics in Enhancing the Immune System
The healthy benefits from fermented foods including yogurt have been recognized for a long time (80). Regular consumption of yogurt replenishes beneficial bacteria and macronutrients that enhance immunity by specifically boosting cell immunity, while downregulating oxidative stress (81, 82). Yogurt fortification with probiotics has been shown to greatly enhance the beneficial effects from a health perspective (83, 84). Typically, probiotic bacteria can survive to extreme conditions in the GI tract and maintain their functional benefits where other bacterial strains are catabolized (85). There are many probiotic microbes each with unique biological features, including therapeutic significance. For instance, some probiotic strains are effective in enhancing GI regularity and improving lactose tolerance, whereas others beyond this review’s scope have been shown to prevent acute upper respiratory tract infections and bacterial vaginosis. Probiotics within the GI tract inhibit both pathogenic microbes adherence and proliferation (83, 86). This is important in immune homeostasis, since probiotic supplements rejuvenate immunity by balancing the gut microbiome, ultimately stabilizing TREG and Th17 cells (87, 88). Moreover, it can be hypothesized that probiotics consumption can potentially dampen the cytokine storm in combination with appropriate antiviral regimens. Despite the gut microbiota’s susceptibility to viral perturbation, it can be equilibrated by dietary interventions. Specifically, to what concerns to COVID-19, gastroenteritis and respiratory distress are some of the most common symptoms (89). By hosting the bulk of body’s lymphoid tissues, the GI tract largely influences the immune function when its integrity is altered (90). To this effect, both pre and probiotics able to equilibrate GI microbiota have been reported to reduce the risk of infection (91).
Probiotics have been shown to neutralize inflammation by inhibiting NK cells activation and equilibrating the GI barrier and gut microbiome. However, the exact mechanisms through which probiotics modulate immunity are not perfectly understood (92, 93). Lactobacillus casei Shirota (LcS) is a widely studied probiotic with immunomodulatory effects (94), being able to enhance immune system against complications from post-operative infection (95), cancer (96), allergies (97), and to regulate GI functions (98). This probiotic boosts the beneficial gut’s bacteria species, augments NK cell activity while maintaining a proper balance between harmful and beneficial bacteria (99, 100). Furthermore, experimental evidence has shown that LcS promotes IL-12 production by peripheral blood mononuclear cells (PBMC) from healthy humans and enhance NK cells’ activity, while heat-inactivated LcS boost the cytokines production, including IL-10, 12, IFN-γ, and TNF-α that enhances CD69 expression in NK cells (94).
Though the synergy between microbiome, fermentation by-products and immune system is not fully known, antigen presentation by dendritic cells is thought to be a key player in this aspect. Thus, dendritic cells are significant immunomodulators by initiating diverse responses to various immune stimulations (101). Dendritic cells from monocytes lineage can also be modulated when they interact with lactic acid bacteria (LAB). This response characterizes infection by bacteria and cell debris. Furthermore, through action of GI fermentative bacteria, other bioactive metabolites with immunomodulatory properties are produced. For example, bioactive products generated by Bifidobacterium breve can modulate cytokines production by intestinal epithelial cells. Indeed, B. breve triggers milk serum fermentation and forms a supernatant that stimulates both dendritic cells development and activation (101).
The Role of Prebiotic in Enhancing the Immune System
Prebiotic is another additive on fermented dairy products with immune enhancing potential. Prebiotics are non-digestible food supplements that potentially stimulate development of gut domiciled bacteria with host’s health benefits (102). For example, the yeast cell walls metabolism generates mannan oligosaccharide (MOS) that contains 30% glucan, 30% mannan, and 12.5% mannoproteins (103, 104). The MOS ability to bind lectin on pathogenic microbes, such as Salmonellaspp and Escherichia coli, minimizes their intestinal proliferation (105, 106).
Another prebiotic yielded as a by-product of fungal/yeast cell wall catabolism is β-glucan, that derives from D-glucose monomers, and are formed via 1–3 β-glycosidic bonds, while the long side chains have a 1–6 glycosidic bond. β-glucans stimulate sentinel cells to produce cytokines and induce lymphocyte multiplication (107). The three types of lymphocytes include NK, T, and B cells. NK and T cells are involved in innate and adaptive immune responses, respectively, whereas B cells are mainly involved in immunoglobulins production. β-glucan can exert its immunomodulatory potential on three lymphocyte categories.
Fructan is another prebiotic derived from plant polysaccharides hydrolyzation or as a by-product of microbial catabolism of plant polysaccharides. The three classes of fructans include levan, inulin and branched groups. Fructan’s biological significance is attributed to its β-glycosidic bond that withstands catabolic activities of digestive enzymes whilst augmenting the levels of commensal bacteria including Lactobacilli and Bifidobacteria spp., whereas pathogenic microbes such as E. coli and Clostridium pefringens are inhibited (41, 108, 109). Other oligosaccharides with prebiotic potency include xylo-oligosaccharides, chitosan, galactoglucomannan, and galacto-oligosaccharides, with studies revealing that they have immunomodulatory activity following supplementation with fermented dairy products, acting on both cytokines and immunoglobulins production, and promoting phagocytosis, NK and T cells’ activity (110, 111). In conclusion, fortification of fermented dairy products with pre- and probiotics could be beneficial in boosting immune system against infections, possibly including SARS-CoV-2.
Experimental Evidences
A study utilizing fecal samples from Polymerase Chain Reaction (PCR) confirmed and hospitalized COVID-19 patients demonstrated presence of more opportunistic pathogens while the commensal microbes in the gut were depleted compared to healthy individuals. The samples from healthy individuals had prevalence of Eubacterium, Roseburia, Lachnospiraceae, Facecalibacterium prausnitzii. Currently, three registered clinical trials are investigating the effect of pre and probiotics on COVID-19 patients. One of the studies is evaluating the beneficial effects of Lactobacillus coryniformison COVID-19 incidence amongst health care workers with a high risk of SARS-CoV2 (NCT 04366180). Another registered clinical trial (NCT04368351) is focusing on bacteriotherapy effects in the treatment of patients with acute diarrhea, and in preventing intensive care in COVID-19 patients. Lastly, the third clinical trial (NCT04366089) is evaluating the adjuvant uses of oxygen-ozone therapy along with probiotic supplementation in COVID-19 patients. Based on these ideas, both pre and probiotics could be proposed as potential tools to be included in the nutritional treatment of COVID-19 patients. Some of these potentially therapeutic probiotics include Lactobacillus rhamnosus and Bifidobacterium lactis, that exhibits anti-inflammatory effects while also rejuvenating both innate and adaptive immunity.
Cheese
Cheese is a milk-derived product, rich in nutrients, such as fats, proteins, essential minerals and vitamins, processed by souring milk or clotting it with renin (112). Cheese processing leads to the formation of a versatile food product with a variety of flavors, textures, and nutritional benefits. Physical features of cheese are influenced by casein content, type, quantity, and their interactional strength, its proximate composition and ripening forms (113).
Nutritional Value of Cheese
Cheese has high nutritive value, being rich in proteins, fat, vitamins, and minerals like calcium (Ca2+) and phosphorous (114). Save for cysteine and methionine, cheese is rich in all essential amino acids enough for recommended human intake (115). Besides being significant in human nutrition, proteins are currently considered as important sources of bioactive peptides. These are amino acid sequences that give to a modified protein its characteristic biological activity. These bioactive peptides exert their actions through downregulating blood pressure, chelating minerals, exerting antimicrobial effects, modifying immune system, reducing inflammation, and cholesterol levels (116).
Cheese as an Immunity Enhancing Product
Studies have shown association between low protein levels (especially immunoglobulins) from cheese and an enhanced risk of infection (114). These nutritional supplements are important in regulating inflammation and oxidative stress responses both known to influence immunity (117). The nutritional impact on immune response is a key aspect in the formulation of anti-inflammatory dietary index (117). For example, dietary supplements rich in omega-3 fatty acids are known to have excellent antioxidant and anti-inflammatory effects, and also a good immunomodulatory potency (118). Elsewhere, experimental protein feeding below 0.8 g/kg body weight in mice, predisposed the animals to severe influenza infection, due to insufficient antibody response, persistence lung viremia, and elevated inflammation with fatal outcomes (119), whereas cheese-derived dietary products rich in calories and saturated fats inhibits inflammation and lipogenesis (120). Thus, the dietary incorporation cheese can reduce post-prandial lipogenesis and inflammatory activities [60]. Furthermore, it has been stated that protein meals, rich in essential amino acids can modulate post-meal glycemic stimuli and improve satiety, since they have better gastric retention and prolonged GI transit (121). This underscores the significance of high quality cheese-derived nutrients, such as calories and saturated fats, as anti-inflammatory enhancing diets that can be incorporated in nutritional immune modulation (122).
Milk
Milk is one of the main dietary sources of protein for humans, and is primarily composed of water (87%), lactose (5%), fats (0.3%), proteins (4%), vitamins (0.1%), and minerals (123). Milk protein can be categorized as either insoluble (casein) or soluble (whey), with latter constituting 20% of milk proteins, while insoluble proteins constitute the remaining fraction (80%) (124). Both protein categories can sufficiently meet the human’s amino acid requirements, besides being digestible and bioavailable. In newborns, milk forms the primary source of bioactive molecules, acting on immune system enhancement against bacteria and viruses and promoting the development of both GI system and bones. The antiviral activity of milk has been attributed to bioactive components with immunomodulatory and anti-inflammatory potential, such as casein, whey proteins and associated peptides (41).
Dietetic Benefits of Milk
Milk is rich in nutritional components essential for a healthy growth and human development. Minerals, such as calcium is vital in regulating high blood pressure besides being crucial in teeth and bones formation, whereas selenium is a key antioxidant that boosts immune system. The active biological compounds present in milk, including essential amino acids and fatty acids makes it superior to meat in terms of biological significance and value. A good example of these fatty acids is omega-3 which has been implicated in inhibiting some types of cancer and CVD. Caseins, mainly classified as alpha, beta and kappa, act by chelating minerals, such as phosphorus and calcium thereby transporting them as a coagulum in the gut (125).
Recent studies have proposed a correlation between beta casein protein components and human health (126, 127). Bovine milk is an ideal protein source containing all essential amino acids, and high levels of branched chain amino acids, thereby considered as a reference baseline for quantifying other proteins with huge nutritive value (3). Branched chain amino acids, particularly leucine, have been indicated in enhancing synthesis of muscle proteins. Whey protein is also rich in methionine and sulfur-containing amino acids, crucial for glutathione formation, to whom great antioxidant and immunomodulatory effects have been attributed, besides being effective against cancer cells (4, 99).
Casein Proteins
Casein is a heterogenous protein family, mainly composed of calcium-phosphate micelle complexes (97), classified into alpha-, beta-, gamma-, and kappa-casein (128, 129). Casein and its derivative peptides are known to modulate immune responses, ultimately enhancing the antiviral activity while mitigating sepsis by immune downregulation (126, 130). Caseins also activate B and T-cell mediated responses, thereby linking innate to adaptive immunity. Several casein-derived peptides are also known to have human health benefits by stimulating CV, digestive, immune and central nervous (CNS) systems. For example, some peptides have shown good antioxidant (131), cytoprotective, immunomodulatory (126), antithrombotic and anti-hypertensive effects (131). An example of a peptide that interacts with the CNS is β-casomorphine that exert analgesic effects by acting like opioids (132). Studies have also demonstrated peptides’ gut interference via enhanced mucin production, thereby inhibiting the pathogens adherence while enhancing the GI retention which has been linked to a better weight management, through regulated food intake (133).
Whey Proteins
Whey proteins are specific high-energy crude nutrients with various textures and thicknesses. They often accompany other palatable foods provided to hospitalized patients to improve protein-calorie intake. In addition, they have been associated with immunomodulatory benefits besides complementing antiviral regimens in patients under medication (134, 135).
Whey proteins found in milk are soluble heat labile globular structured compounds stabilized by intermolecular disulfide linkages. Being a heterogenous protein, it is composed of polymorphic proteins including beta-lactoglobulin and alpha-lactalbumin that confers the characteristic foaming and gelation properties (124, 136). Proteose, peptone, immunoglobulin, and glycomacropeptide found in whey have also shown in vitro and in vivo bioactivities (137). Other whey constituents include lactoferrin, bovine serum albumin (BSA), lactoperoxidase, non-protein components, such as vitamins, fats, lactose, and minerals (138, 139). Lactoferrin is an important immunomodulator with interesting antimicrobial and antioxidant effects. Animal and human experiments have even shown that oral lactoferrin administration is effective against infections, cancer and inflammation, thereby making it an appropriate food additive (140).
Immune System Enhancement by Whey Proteins
Experimental evidence has demonstrated that whey proteins have huge immune response enhancing properties. For instance, whey was able to elevate mucosal antibody response against cholera toxin and ovalbumin when fed to mice for 12 weeks compared to control on a regular diet (141). In another study, bovine whey proteins consumption (for 5–8 weeks) by mice led to a marked raise on foot pad delayed-type hypersensitivity responses and in vitro concanavalin A-induced spleen cell proliferation (141). The helper T cell (L3T4+) populations were also elevated in mice receiving undenatured whey protein (25 g) for 4 weeks compared to the control group fed an isocaloric casein diet (142). Not only the L3T4+ cells population were elevated but also the helper (L3T4+) to suppressor T-cells (Lyt-2+) ratio too. In comparison to casein and soy protein rich diets, whey protein-diet has been linked to an elevated count of CD4+, and C8+ lymphocytes, total white blood cells and increased IFN-γ production by concanavalin A-stimulated spleen in experimental mice (143). Whey proteins also increase the glutathione levels in plasma and enhance the NK cells activity in patients with chronic hepatitis B (144). Moreover, whey proteins’ antiviral activity has been reported against HIV. Further, β-LF strongly inhibits reverse transcriptase and mildly inhibits integrase and protease, whereas β-LG and α-LA inhibits integrase and protease but not reverse transcriptase during the early infection stages (145). Elsewhere, whey derivatives, such as β-lactoglobulin and α-lactalbumin have been shown to bind and deter attachment of rotavirus to the host’s cells receptors (146). In addition, lactoperoxidase enzyme from whey when combined with thiocyanate and hydrogen peroxide substrates also exhibits antiviral activity against herpes, HIV and echovirus (147, 148). Moreover, when orally administered, lactoperoxidase can mediate pneumonia disruption in mice experimentally infected with influenza by suppressing inflammation in lung cells (149).
Conclusion
SARS-CoV-2 infection has triggered a serious global devastation in human being’s well-being and health maintenance, and a huge impact in healthcare delivery systems. Elderly individuals are particularly vulnerable to such infection, as both physiological and immunological performance declines with age, ultimately raising the risk of refractory infections and gets serious ill. Hence, implementation of infection control programs is difficult for them. This review mostly aimed to clarify the value of systemic dietary distortions in COVID-19 patients, as immunity is impaired by a poor nutritional status. For that purpose, here the different types of food that enhance humans’ immune response, such as egg-derived proteins, milk, and fermented dairy products containing different probiotics were addressed and discussed. Eggs, yogurt, cheese, and milk are rich sources of essential macro and micronutrients, that possess excellent immunomodulatory, anti-inflammatory, antioxidant and antiviral activities, being thus essential for the maintenance of a healthy growth and development.
Author Contributions
GB, DA, AA, RN, MW, MS, MNA, BA, NC-M, NK, HH, AG, and J-MS wrote and carefully revised the paper. All authors have read and agreed to the published version of the manuscript.
Conflict of Interest
The authors declare that the research was conducted in the absence of any commercial or financial relationships that could be construed as a potential conflict of interest.
Acknowledgments
NC-M acknowledges the Portuguese Foundation for Science and Technology under the Horizon 2020 Program (PTDC/PSI-GER/28076/2017). Figures 1, 2 were made using Biorender.com.
References
1. Weston S, Frieman M. COVID-19: knowns, unknowns, and questions. mSphere. (2020) 5:e00203-20. doi: 10.1128/mSphere.00203-20
2. Lake MA. What we know so far: COVID-19 current clinical knowledge and research. Clin Med. (2020) 20:124. doi: 10.7861/clinmed.2019-coron
3. Rothan HA, Byrareddy SN. The epidemiology and pathogenesis of coronavirus disease (COVID-19) outbreak. J Autoimmun. 2020:102433 .doi: 10.1016/j.jaut.2020.102433
4. Phelan AL, Katz R, Gostin LO. The novel coronavirus originating in Wuhan, China: challenges for global health governance. Jama.(2020) 323:709–10. doi: 10.1001/jama.2020.1097
5. Wang C, Horby PW, Hayden FG, Gao GF. A novel coronavirus outbreak of global health concern. Lancet. (2020) 395:470–3. doi: 10.1016/S0140-6736(20)30185-9
6. Alwarawrah Y, Kiernan K, MacIver NJ. Changes in nutritional status impact immune cell metabolism and function. Front Immunol.(2018) 9:1055. doi: 10.3389/fimmu.2018.01055
7. Yaqoob P. Ageing alters the impact of nutrition on immune function. Proc Nutr Soc. (2017) 76:347–51. doi: 10.1017/S0029665116000781
8. Lomax AR, Calder PC. Prebiotics, immune function, infection and inflammation: a review of the evidence. Br J Nutr. (2008) 101:633–58. doi: 10.1017/S0007114508055608
9. Villani A-C, Sarkizova S, Hacohen N. Systems immunology: learning the rules of the immune system. Ann Rev Immunol. (2018) 36:813–42. doi: 10.1146/annurev-immunol-042617-053035
10. Routy J-P, Mehraj V, Cao W. HIV Immunotherapy Comes of Age: Implications for Prevention, Treatment and Cure. Abingdon: Taylor & Francis (2016). doi: 10.1586/1744666X.2016.1112269
11. Naidoo J, Page D, Wolchok J. Immune modulation for cancer therapy. Br J Cancer. (2014) 111:2214–9. doi: 10.1038/bjc.2014.348
12. Ozkan MC, Tombuloglu M, Sahin F, Saydam G. Evaluation of immunomodulatory drugs in multiple myeloma: single center experience. Am J Blood Res. (2015) 5:95.
13. Flores C, Fouquet G, Moura IC, Maciel TT, Hermine O. Lessons to learn from low-dose cyclosporin-A: a new approach for unexpected clinical applications. Front Immunol. (2019) 10:588. doi: 10.3389/fimmu.2019.00588
14. Gong Y, Klingenberg SL, Gluud C. D-penicillamine for primary biliary cirrhosis. Cochr Datab Syst Rev. (2004) 18:CD004789. doi: 10.1002/14651858.CD004789.pub2
15. Ahlmann M, Hempel G. The effect of cyclophosphamide on the immune system: implications for clinical cancer therapy. Cancer Chemother Pharmacol. (2016) 78:661–71. doi: 10.1007/s00280-016-3152-1
16. Ulrich C, Bichel J, Euvrard S, Guidi B, Proby C, Van De Kerkhof P, et al. Topical immunomodulation under systemic immunosuppression: results of a multicentre, randomized, placebo-controlled safety and efficacy study of imiquimod 5% cream for the treatment of actinic keratoses in kidney, heart, and liver transplant patients. Br J Dermatol. (2007) 157:25–31. doi: 10.1111/j.1365-2133.2007.08269.x
17. Turvey SE, Broide DH. Innate immunity. J Allergy Clin Immunol. (2010) 125:S24–32. doi: 10.1016/j.jaci.2009.07.016
18. Raval RR, Sharabi AB, Walker AJ, Drake CG, Sharma P. Tumor immunology and cancer immunotherapy: summary of the (2013). SITC primer. J Immunother Cancer. (2014) 2:14. doi: 10.1186/2051-1426-2-14
19. Arango Duque G, Descoteaux A. Macrophage cytokines: involvement in immunity and infectious diseases. Front Immunol. (2014) 5:491. doi: 10.3389/fimmu.2014.00491
20. Lim YT. Vaccine adjuvant materials for cancer immunotherapy and control of infectious disease. Clin Exp Vaccine Res. (2015) 4:54–8. doi: 10.7774/cevr.2015.4.1.54
21. Broere F, van Eden W. T cell subsets and T cell-mediated immunity. In: Parnham M, Nijkamp F, Rossi A, editors. Nijkamp and Parnham’s Principles of Immunopharmacology. Cham: Springer (2019). p. 23–35. doi: 10.1007/978-3-030-10811-3_3
22. Parnham MJ, Nijkamp FP. Principles of Immunopharmacology. Basel: Springer (2005). doi: 10.1007/3-7643-7408-X
23. Pan M-H, Lai C-S, Ho C-T. Anti-inflammatory activity of natural dietary flavonoids. Food Funct. (2010) 1:15–31. doi: 10.1039/c0fo00103a
24. Zeng C, Zhong P, Zhao Y, Kanchana K, Zhang Y, Khan ZA, et al. Curcumin protects hearts from FFA-induced injury by activating Nrf2 and inactivating NF-κB both in vitro and in vivo. J Mol Cell Cardiol. (2015) 79:1–12. doi: 10.1016/j.yjmcc.2014.10.002
25. Siriwardhana N, Kalupahana NS, Cekanova M, LeMieux M, Greer B, Moustaid-Moussa N. Modulation of adipose tissue inflammation by bioactive food compounds. J Nutr Biochem. (2013) 24:613–23. doi: 10.1016/j.jnutbio.2012.12.013
26. Herron KL, Fernandez ML. Are the current dietary guidelines regarding egg consumption appropriate? J Nutr. (2004) 134:187–90. doi: 10.1093/jn/134.1.187
27. Millward DJ, Layman DK, Tomé D, Schaafsma G. Protein quality assessment: impact of expanding understanding of protein and amino acid needs for optimal health. Am J Clin Nutr. (2008) 87:1576S−81S. doi: 10.1093/ajcn/87.5.1576S
28. Moore DR, Robinson MJ, Fry JL, Tang JE, Glover EI, Wilkinson SB, et al. Ingested protein dose response of muscle and albumin protein synthesis after resistance exercise in young men. Am J Clin Nutr. (2009) 89:161–8. doi: 10.3945/ajcn.2008.26401
29. Cotterill O, Geiger G. Egg product yield trends from shell eggs. Poult Sci. (1977) 56:1027–31. doi: 10.3382/ps.0561027
30. Ridgman W. The Avian Egg. Chemistry and Biology, by RW Burley & DV Vadehra. v+ 472 pp. New York: Wiley. (1989) £ 66.70 (hard covers). ISBN 0 471 84995 2. J Agric Sci. (1991) 116:169–70. doi: 10.1017/S0021859600076310
31. Li-Chan EC. The chemistry of eggs and egg products. In: Eunice C, Li-Chan Y, Powrie WD, Nakai S, editors. Egg Science and Technology. Boca Raton, FL: CRC Press (1995). p. 105–75.
32. Juneja LR, Yamamoto T, Hatta H, Kim M. Hen Eggs: Their Basic and Applied Science. Boca Raton, FL: CRC Press (1997).
33. Mine Y. Recent advances in egg protein functionality in the food system. World’s Poult Sci J. (2002) 58:31–9. doi: 10.1079/WPS20020005
34. Børsheim E, Tipton KD, Wolf SE, Wolfe RR. Essential amino acids and muscle protein recovery from resistance exercise. Am J Physiol Endocrinol Metab. (2002) 283:E648–57. doi: 10.1152/ajpendo.00466.2001
35. US Department of Agriculture ARS. USDA national nutrient database for standard reference, release 28. Nutrient data laboratory home page (2011).
36. Kovacs-Nolan J, Phillips M, Mine Y. Advances in the value of eggs and egg components for human health. J Agric Food Chem. (2005) 53:8421–31. doi: 10.1021/jf050964f
37. Miranda JM, Anton X, Redondo-Valbuena C, Roca-Saavedra P, Rodriguez JA, Lamas A, et al. Egg and egg-derived foods: effects on human health and use as functional foods. Nutrients. (2015) 7:706–29. doi: 10.3390/nu7010706
38. Kuang H, Yang F, Zhang Y, Wang T, Chen G. The impact of egg nutrient composition and its consumption on cholesterol homeostasis. Cholesterol. (2018) 2018:6303810. doi: 10.1155/2018/6303810
39. Jung S, Kim DH, Son JH, Nam K, Ahn DU, Jo C. The functional property of egg yolk phosvitin as a melanogenesis inhibitor. Food Chem. (2012) 135:993–8. doi: 10.1016/j.foodchem.2012.05.113
40. Natoli S, Markovic T, Lim D, Noakes M, Kostner K. Unscrambling the research: eggs, serum cholesterol andcoronary heart disease. Nutr Diet. (2007) 64:105–11. doi: 10.1111/j.1747-0080.2007.00093.x
41. Kim G-B, Seo Y, Kim C, Paik I. Effect of dietary prebiotic supplementation on the performance, intestinal microflora, and immune response of broilers. Poult Sci. (2011) 90:75–82. doi: 10.3382/ps.2010-00732
42. Kobayashi Y, Rupa P, Kovacs-Nolan J, Turner PV, Matsui T, Mine Y. Oral administration of hen egg white ovotransferrin attenuates the development of colitis induced by dextran sodium sulfate in mice. J Agric Food Chem. (2015) 63:1532–9. doi: 10.1021/jf505248n
43. Yokooji T, Hamura K, Matsuo H. Intestinal absorption of lysozyme, an egg-white allergen, in rats: kinetics and effect of NSAIDs. Biochem Biophys Res Commun. (2013) 438:61–5. doi: 10.1016/j.bbrc.2013.07.024
44. Yokooji T, Nouma H, Matsuo H. Characterization of ovalbumin absorption pathways in the rat intestine, including the effects of aspirin. Biol Pharm Bull. (2014) 37:1359–65. doi: 10.1248/bpb.b14-00290
45. Medzhitov R. Origin and physiological roles of inflammation. Nature. (2008) 454:428–35. doi: 10.1038/nature07201
46. Medzhitov R. Recognition of microorganisms and activation of the immune response. Nature. (2007) 449:819–26. doi: 10.1038/nature06246
47. Rupa P, Schnarr L, Mine Y. Effect of heat denaturation of egg white proteins ovalbumin and ovomucoid on CD4+ T cell cytokine production and human mast cell histamine production. J Funct Foods. (2015) 18:28–34. doi: 10.1016/j.jff.2015.06.030
48. Lee JH, Ahn DU, Paik H-D. In vitro immune-enhancing activity of ovotransferrin from egg white via MAPK signaling pathways in RAW 264.7 macrophages. Korean J Food Sci Anim Resour. (2018) 38:1226. doi: 10.5851/kosfa.2018.e56
49. Sun X, Chakrabarti S, Fang J, Yin Y, Wu J. Low-molecular-weight fractions of Alcalase hydrolyzed egg ovomucin extract exert anti-inflammatory activity in human dermal fibroblasts through the inhibition of tumor necrosis factor–mediated nuclear factor κB pathway. Nutr Res. (2016) 36:648–57. doi: 10.1016/j.nutres.2016.03.006
50. Vidovic D, Graddis T, Chen F, Slagle P, Diegel M, Stepan L, et al. Antitumor vaccination with HER-2-derived recombinant antigens. Int J Cancer. (2002) 102:660–4. doi: 10.1002/ijc.10785
51. Pellegrini A, Thomas U, Wild P, Schraner E, Von Fellenberg R. Effect of lysozyme or modified lysozyme fragments on DNA and RNA synthesis and membrane permeability of Escherichia coli. Microbiol Res. (2000) 155:69–77. doi: 10.1016/S0944-5013(00)80040-3
52. Meram C, Wu J. Anti-inflammatory effects of egg yolk livetins (α, β, and γ-livetin) fraction and its enzymatic hydrolysates in lipopolysaccharide-induced RAW 264.7 macrophages. Food Res Int. (2017) 100:449–59. doi: 10.1016/j.foodres.2017.07.032
53. He X, Tsang TC, Luo P, Zhang T, Harris DT. Enhanced tumor immunogenicitythrough coupling cytokine expression with antigen presentation. Cancer Gene Ther. (2003) 10:669–77. doi: 10.1038/sj.cgt.7700618
54. Ha Y-m, Chun S-H, Hong S-t, Koo Y-c, Choi H-d, Lee K-w. Immune enhancing effect of a Maillard-type lysozyme-galactomannan conjugate via signaling pathways. Int J Biol Macromol. (2013) 60:399–404 .doi: 10.1016/j.ijbiomac.2013.06.007
55. Sava G. Pharmacological aspects and therapeutic applications of lysozymes. EXS. (1996) 75:433–49. doi: 10.1007/978-3-0348-9225-4_22
56. Fan X, Subramaniam R, Weiss M, Monnier V. Methylglyoxal–bovine serum albumin stimulates tumor necrosis factor alpha secretion in RAW 264.7 cells through activation of mitogen-activating protein kinase, nuclear factor κB and intracellular reactive oxygen species formation. Arch Biochem Biophys. (2003) 409:274–86. doi: 10.1016/S0003-9861(02)00599-4
57. Mine Y, D’Silva I. Bioactive components in egg white. In: Mine Y, editor. Egg Bioscience and Biotechnology. New York, NY: John Wiley & Sons (2008). p. 141–84. doi: 10.1002/9780470181249.ch4
58. Goldberg J, Shrikant P, Mescher MF. In vivo augmentation of tumor-specific CTL responses by class I/peptide antigen complexes on microspheres (large multivalent immunogen). J Immunol. (2003) 170:228–35. doi: 10.4049/jimmunol.170.1.228
59. Xie H, Huff GR, Huff WE, Balog JM, Rath NC. Effects of ovotransferrin on chicken macrophages and heterophil-granulocytes. Dev Comp Immunol. (2002) 26:805–15. doi: 10.1016/S0145-305X(02)00028-9
60. Tanizaki H, Tanaka H, Iwata H, Kato A. Activation of macrophages by sulfated glycopeptides in ovomucin, yolk membrane, and chalazae in chicken eggs. Biosci Biotechnol Biochem. (1997) 61:1883–9. doi: 10.1271/bbb.61.1883
61. Verdot L, Lalmanach G, Vercruysse V, Hoebeke J, Gauthier F, Vray B. Chicken cystatin stimulates nitric oxide release from interferon-γ-activated mouse peritoneal macrophages via cytokine synthesis. Eur J Biochem. (1999) 266:1111–7. doi: 10.1046/j.1432-1327.1999.00964.x
62. Kato T, Imatani T, Miura T, Minaguchi K, Saitoh E, Okuda K. Cytokine-inducing activity of family 2 cystatins. Biol Chem. (2000) 381:1143–7. doi: 10.1515/BC.2000.141
63. Sugahara T, Murakami F, Yamada Y, Sasaki T. The mode of actions of lysozyme as an immunoglobulin production stimulating factor. Biochim Biophys Acta. (2000) 1475:27–34. doi: 10.1016/S0304-4165(00)00041-6
64. Asakura K, Kojima T, Shirasaki H, Kataura A. Evaluation of the effects of antigen specific immunotherapy on chronic sinusitis in children with allergy. Auris Nasus Larynx. (1990) 17:33–8. doi: 10.1016/S0385-8146(12)80018-6
65. Shiby V, Mishra H. Fermented milks and milk products as functional foods—a review. Crit Rev Food Sci Nutr. (2013) 53:482–96. doi: 10.1080/10408398.2010.547398
66. Brandtzaeg P. Current understanding of gastrointestinal immunoregulation and its relation to food allergy. Ann N Y Acad Sci. (2002) 964:13–45. doi: 10.1111/j.1749-6632.2002.tb04131.x
67. Rhee K-J, Sethupathi P, Driks A, Lanning DK, Knight KL. Role of commensal bacteria in development of gut-associated lymphoid tissues and preimmune antibody repertoire. J Immunol. (2004) 172:1118–24. doi: 10.4049/jimmunol.172.2.1118
68. Oskar A, Meydani S, Russell R. Yogurt and gut function. Am J Clin Nutr. (2004) 80:245–56. doi: 10.1093/ajcn/80.2.245
69. Kerry RG, Patra JK, Gouda S, Park Y, Shin H-S, Das G. Benefaction of probiotics for human health: a review. J Food Drug Anal. (2018) 26:927–39. doi: 10.1016/j.jfda.2018.01.002
70. Meydani SN, Ha W-K. Immunologic effects of yogurt. Am J Clin Nutr. (2000) 71:861–72. doi: 10.1093/ajcn/71.4.861
71. Hooper LV, Littman DR, Macpherson AJ. Interactions between the microbiota and the immune system. Science. (2012) 336:1268–73. doi: 10.1126/science.1223490
72. Pan L, Mu M, Yang P, Sun Y, Wang R, Yan J, et al. Clinical characteristics of COVID-19 patients with digestive symptoms in Hubei, China: a descriptive, cross-sectional, multicenter study. Am J Gastroenterol. (2020) 115:620. doi: 10.14309/ajg.0000000000000620
73. Guan W-j, Ni Z-y, Hu Y, Liang W-h, Ou C-q, He J-x, et al. Clinical characteristics of coronavirus disease 2019 in China. New Eng J Med.(2020) 382:1708–20. doi: 10.1056/NEJMoa2002032
74. Wang T, Du Z, Zhu F, Cao Z, An Y, Gao Y, et al. Comorbidities and multi-organ injuries in the treatment of COVID-19. Lancet. (2020) 395:e52. doi: 10.1016/S0140-6736(20)30558-4
75. Galdeano CM, Perdigon G. Role of viability of probiotic strains in their persistence in the gut and in mucosal immune stimulation. J Appl Microbiol. (2004) 97:673–81. doi: 10.1111/j.1365-2672.2004.02353.x
76. Talwalkar A, Kailasapathy K. A review of oxygen toxicity in probiotic yogurts: influence on the survival of probiotic bacteria and protective techniques. Comp Rev Food Sci Food Safety. (2004) 3:117–24. doi: 10.1111/j.1541-4337.2004.tb00061.x
77. Hosono A, Otani H, Yasui H, Watanuki M. Impact of fermented milk on human health: cholesterol-lowering and immunomodulatory properties of fermented milk. Anim Sci J. (2002) 73:241–56. doi: 10.1046/j.1344-3941.2002.00034.x
78. Boza-Méndez E, López-Calvo R, Cortés-Muñoz M. Innovative dairy products development using probiotics: challenges and limitations. In Tech Costarica. (2012) 10:213–36. doi: 10.5772/50104
79. Lourens-Hattingh A, Viljoen BC. Yogurt as probiotic carrier food. Int Dairy J. (2001) 11:1–17. doi: 10.1016/S0958-6946(01)00036-X
80. Oyewole OB. Lactic fermented foods in Africa and their benefits. Food Control. (1997) 8:289–97. doi: 10.1016/S0956-7135(97)00075-3
81. Meyer AL, Micksche M, Herbacek I, Elmadfa I. Daily intake of probiotic as well as conventional yogurt has a stimulating effect on cellular immunity in young healthy women. Ann Nutr Metab. (2006) 50:282–9. doi: 10.1159/000091687
82. Sanders ME. Use of probiotics and yogurts in maintenance of health. J Clin Gastroenterol. (2008) 42:S71–4. doi: 10.1097/MCG.0b013e3181621e87
83. Anukam KC, Osazuwa EO, Osadolor HB, Bruce AW, Reid G. Yogurt containing probiotic Lactobacillus rhamnosus GR-1 and L. reuteriRC-14 helps resolve moderate diarrhea and increases CD4 count in HIV/AIDS patients. J Clin Gastroenterol. (2008) 42:239–43. doi: 10.1097/MCG.0b013e31802c7465
84. Reid G. The importance of guidelines in the development and application of probiotics. Curr Pharm Des. (2005) 11:11–6. doi: 10.2174/1381612053382395
85. Tuomola E, Crittenden R, Playne M, Isolauri E, Salminen S. Quality assurance criteria for probiotic bacteria. Am J Clin Nutr. (2001) 73:393–8. doi: 10.1093/ajcn/73.2.393s
86. Canani RB, Cirillo P, Terrin G, Cesarano L, Spagnuolo MI, De Vincenzo A, et al. Probiotics for treatment of acute diarrhoea in children: randomised clinical trial of five different preparations. Bmj. (2007) 335:340. doi: 10.1136/bmj.39272.581736.55
87. Wu D, Yang XO. TH17 responses in cytokine storm of COVID-19: an emerging target of JAK2 inhibitor Fedratinib. J Microbiol Immunol Infect. (2020) 53:368–70. doi: 10.1016/j.jmii.2020.03.005
88. De Lorenzo A, Costacurta M, Merra G, Gualtieri P, Cioccoloni G, Marchetti M, et al. Can psychobiotics intake modulate psychological profile and body composition of women affected by normal weight obese syndrome and obesity? A double blind randomized clinical trial. J Transl Med. (2017) 15:135. doi: 10.1186/s12967-017-1236-2
89. Ferrey AJ, Choi G, Hanna RM, Chang Y, Tantisattamo E, Ivaturi K, et al. A case of novel coronavirus disease 19 in a chronic hemodialysis patient presenting with gastroenteritis and developing severe pulmonary disease. Am J Nephrol. (2020) 51:337–42. doi: 10.1159/000507417
90. Dandekar S. Pathogenesis of HIV in the gastrointestinal tract. Curr HIV/AIDS Rep. (2007) 4:10–5. doi: 10.1007/s11904-007-0002-0
91. Yang H, Sun Y, Cai R, Chen Y, Gu B. The impact of dietary fiber and probiotics in infectious diseases. Microb Pathog. (2020) 140:103931. doi: 10.1016/j.micpath.2019.103931
92. Gori A, Rizzardini G, Van’t Land B, Amor KB, Van Schaik J, Torti C, et al. Specific prebiotics modulate gut microbiota and immune activation in HAART-naive HIV-infected adults: results of the “COPA” pilot randomized trial. Mucosal Immunol. (2011) 4:554–63. doi: 10.1038/mi.2011.15
93. Kekkonen RA, Lummela N, Karjalainen H, Latvala S, Tynkkynen S, Järvenpää S, et al. Probiotic intervention has strain-specific anti-inflammatory effects in healthy adults. World J Gastroenterol. (2008) 14:2029. doi: 10.3748/wjg.14.2029
94. Takeda K, Suzuki T, Shimada SI, Shida K, Nanno M, Okumura K. Interleukin-12 is involved in the enhancement of human natural killer cell activity by Lactobacillus casei Shirota. Clin Exp Immunol. (2006) 146:109–15. doi: 10.1111/j.1365-2249.2006.03165.x
95. Sugawara G, Nagino M, Nishio H, Ebata T, Takagi K, Asahara T, et al. Perioperative synbiotic treatment to prevent postoperative infectious complications in biliary cancer surgery: a randomized controlledtrial. Ann Surg. (2006) 244:706. doi: 10.1097/01.sla.0000219039.20924.88
96. Aso Y, Akaza H, Kotake T, Tsukamoto T, Imai K, Naito S, et al. Preventive effect of a Lactobacillus casei preparation on the recurrence of superficial bladder cancer in a double-blind trial. Eur Urol. (1995) 27:104–9. doi: 10.1159/000475138
97. Tamura M, Shikina T, Morihana T, Hayama M, Kajimoto O, Sakamoto A, et al. Effects of probiotics on allergic rhinitis induced by Japanese cedar pollen: randomized double-blind, placebo-controlled clinical trial. Int Arch Allergy Immunol. (2007) 143:75–82. doi: 10.1159/000098318
98. Shioiri T, Yahagi K, Nakayama S, Asahara T, Yuki N, Kawakami K, et al. The effects of a synbiotic fermented milk beverage containing Lactobacillus casei strain Shirota and transgalactosylated oligosaccharides on defecation frequency, intestinal microflora, organic acid concentrations, and putrefactive metabolites of sub-optimal health state volunteers: a randomized placebo-controlled cross-over study. Biosci Microflora. (2006) 25:137–46. doi: 10.12938/bifidus.25.137
99. Matsumoto K, Takada T, Shimizu K, Moriyama K, Kawakami K, Hirano K, et al. Effects of a probiotic fermented milk beverage containing Lactobacillus casei strain Shirota on defecation frequency, intestinal microbiota, and the intestinal environment of healthy individuals with soft stools. J Biosci Bioeng. (2010) 110:547–52. doi: 10.1016/j.jbiosc.2010.05.016
100. Dong H, Rowland I, Thomas LV, Yaqoob P. Immunomodulatory effects of a probiotic drink containing Lactobacillus casei Shirota in healthy older volunteers. Eur J Nutr. (2013) 52:1853–63. doi: 10.1007/s00394-012-0487-1
101. Granier A, Goulet O, Hoarau C. Fermentation products: immunological effects on human and animal models. Pediatr Res. (2013) 74:238–44. doi: 10.1038/pr.2013.76
102. Roberfroid M, Gibson GR, Hoyles L, McCartney AL, Rastall R, Rowland I, et al. Prebiotic effects: metabolic and health benefits. Br J Nutr.(2010) 104:S1–63. doi: 10.1017/S0007114510003363
103. Baurhoo B, Ferket P, Zhao X. Effects of diets containing different concentrations of mannanoligosaccharide or antibiotics on growth performance, intestinal development, cecal and litter microbial populations, and carcass parameters of broilers. Poult Sci. (2009) 88:2262–72. doi: 10.3382/ps.2008-00562
104. Yang Y, Iji P, Choct M. Dietary modulation of gut microflora in broiler chickens: a review of the role of six kinds of alternatives to in-feed antibiotics. World Poult Sci J. (2009) 65:97–114. doi: 10.1017/S0043933909000087
105. Ofek I, Beachey EH. Mannose binding and epithelial cell adherence of Escherichia coli. Infect Immun. (1978) 22:247–54. doi: 10.1128/iai.22.1.247-254.1978
106. Spring P, Wenk C, Dawson K, Newman K. The effects of dietary mannaoligosaccharides on cecal parameters and the concentrations of enteric bacteria in the ceca of salmonella-challenged broiler chicks. Poult Sci. (2000) 79:205–11. doi: 10.1093/ps/79.2.205
107. Swiatkiewicz S, Arczewska-Włosek A, Józefiak D. Immunomodulatory efficacy of yeast cell products in poultry: a current review. World Poult Sci J. (2014) 70:57–68. doi: 10.1017/S0043933914000051
108. Ricke S. Potential of fructooligosaccharide prebiotics in alternative and nonconventionalpoultry production systems. Poult Sci. (2015) 94:1411–8. doi: 10.3382/ps/pev049
109. Xu Z, Hu C, Xia M, Zhan X, Wang M. Effects of dietary fructooligosaccharide on digestive enzyme activities, intestinal microflora and morphology of male broilers. Poult Sci. (2003) 82:1030–6. doi: 10.1093/ps/82.6.1030
110. Nagao F, Nakayama M, Muto T, Okumura K. Effects of a fermented milk drink containing Lactobacillus casei strain Shirota on the immune system in healthy human subjects. Biosci Biotechnol Biochem. (2000) 64:2706–8. doi: 10.1271/bbb.64.2706
111. Forssten SD, Lahtinen SJ, Ouwehand AC. The intestinal microbiota and probiotics. In: Malago J, Koninkx J, Marinsek-Logar R, editors. Probiotic Bacteria and Enteric Infections. Dordrecht: Springer (2011). p. 41–63. doi: 10.1007/978-94-007-0386-5_2
112. Kress-Rogers E, Brimelow CJ. Instrumentation and Sensors for the Food Industry. Sawston: Woodhead Publishing (2001). doi: 10.1201/9781439833049
113. Banville V, Morin P, Pouliot Y, Britten M. Physical properties of pizza Mozzarella cheese manufactured under different cheese-making conditions. J Dairy Sci. (2013) 96:4804–15. doi: 10.3168/jds.2012-6314
114. Badawi O. Dairy products as source of some bacterial zoonosis. PH. D. thesis. Fac. Vet. Med., Cairo University (1996).
115. Tome D, Bos C, Mariotti F, Gaudichon C. Protein quality and FAO/WHO recommendations. Sci des Alim. (2002) 22:393–405. doi: 10.3166/sda.22.393-405
116. Bachmann H-P, Bütikofer U, Sieber R. Über dasVorkommen von bioaktiven Peptiden in Käse. Mitt Lebensm Hyg. (2003) 94:136–54.
117. Gabriele M, Pucci L. Diet bioactive compounds: implications for oxidative stress and inflammation in the vascular system. Endocr Metab Immune Disord Drug Targets. (2017) 17:264–75. doi: 10.2174/1871530317666170921142055
118. Calder PC. Polyunsaturated fatty acids, inflammatory processes and inflammatory bowel diseases. Mol Nutr Food Res. (2008) 52:885–97. doi: 10.1002/mnfr.200700289
119. Taylor AK, Cao W, Vora KP, Cruz JDL, Shieh W-J, Zaki SR, et al. Protein energy malnutrition decreases immunity and increases susceptibility to influenza infection in mice. J Infect Dis. (2013) 207:501–10. doi: 10.1093/infdis/jis527
120. Jakulj F, Zernicke K, Bacon SL, Van Wielingen LE, Key BL, West SG, et al. A high-fat meal increases cardiovascular reactivity to psychological stress in healthy young adults. J Nutr. (2007) 137:935–9. doi: 10.1093/jn/137.4.935
121. Arora SK, McFarlane SI. The case for low carbohydrate diets in diabetes management. Nutr Metab. (2005) 2:1–9. doi: 10.1186/1743-7075-2-16
122. O’Keefe JH, Gheewala NM, O’Keefe JO. Dietary strategies for improving post-prandial glucose, lipids, inflammation, and cardiovascular health. J Am Coll Cardiol. (2008) 51:249–55. doi: 10.1016/j.jacc.2007.10.016
123. Lindmark-Månsson H, Fondén R, Pettersson H-E. Composition of Swedish dairy milk. Int Dairy J. (2003) 13:409–25. doi: 10.1016/S0958-6946(03)00032-3
124. Walzem R, Dillard C, German JB. Whey components: millennia of evolution create functionalities for mammalian nutrition: what we know and what we may be overlooking. Crit Rev Food Sci Nutr. (2002) 42:353–75. doi: 10.1080/10408690290825574
125. Holt C, Carver J, Ecroyd H, Thorn D. Invited review: caseins and the casein micelle: their biological functions, structures, and behavior in foods. J Dairy Sci. (2013) 96:6127–46. doi: 10.3168/jds.2013-6831
126. Mishra S, Joshi B. Genetic and non-genetic factors affecting lactation milk constituents and yield traits in Holstein Friesian × Karan crossbred cows. Indian J Dairy Sci. (2009) 57:69–72.
127. Sodhi SS, Mehra M, Jain A, Trehan P. Effect of non-genetic factors on the composition of milk of Murrah buffaloes. Indian Vet J. (2008) 85:950–52.
128. Saxelin M, Korpela R, Mäyrä-Mäkinen A. Introduction: classifying functional dairy products. In: Mattila-Sandholm T, Saarela M, editors. Functional Dairy Products. Boca Raton, FL: CRC Press (2003). p. 1–16. doi: 10.1533/9781855736917.1
130. Tellez A, Corredig M, Brovko LY, Griffiths MW. Characterization of immune-active peptides obtained from milk fermented by Lactobacillus helveticus. J Dairy Res. (2010) 77:129–36. doi: 10.1017/S002202990999046X
131. Zimecki M, Kruzel ML. Milk-derived proteins and peptides of potential therapeutic and nutritive value. J Exp Ther Oncol. (2007) 6:89–106.
132. Xia T, Li N, Nel AE. Potential health impact of nanoparticles. Annu Rev Public Health. (2009) 30:137–50. doi: 10.1146/annurev.publhealth.031308.100155
133. De Noni I, Cattaneo S. Occurrence of β-casomorphins 5 and 7 in commercial dairy products and in their digests following in vitrosimulated gastro-intestinal digestion. Food Chem. (2010) 119:560–6. doi: 10.1016/j.foodchem.2009.06.058
134. Ng TB, Cheung RCF, Wong JH, Wang Y, Ip DTM, Wan DCC, et al. Antiviral activities of whey proteins. Appl Microbiol Biotechnol. (2015) 99:6997–7008. doi: 10.1007/s00253-015-6818-4
135. Olsen MF, Abdissa A, Kæstel P, Tesfaye M, Yilma D, Girma T, et al. Effects of nutritional supplementation for HIV patients starting antiretroviral treatment: randomised controlled trial in Ethiopia. Bmj. (2014) 348:g3187. doi: 10.1136/bmj.g3187
136. Séverin S, Wenshui X. Milk biologically active components as nutraceuticals. Crit Rev Food Sci Nutr. (2005) 45:645–56. doi: 10.1080/10408690490911756
137. Hall W, Millward D, Long S, Morgan L. Casein and whey exert different effects on plasma amino acid profiles, gastrointestinal hormone secretion and appetite. Br J Nutr. (2003) 89:239–48. doi: 10.1079/BJN2002760
139. Krissansen GW. Emerging health properties of whey proteins and their clinical implications. J Am Coll Nutr. (2007) 26:713–23. doi: 10.1080/07315724.2007.10719652
140. El-Loly MM, Mahfouz MB. Lactoferrin in relation to biological functions and applications: a review. Int J Dairy Sci. (2011) 6:79–111. doi: 10.3923/ijds.2011.79.111
141. Wong CW, Watson DL. Immunomodulatory effects of dietary whey proteins in mice. J Dairy Res. (1995) 62:359–68. doi: 10.1017/S0022029900031058
142. Baruchel S, Bounous G, Falutz J, Gold P. Whey proteins as a food supplement in HIV-seropositive individuals. Clin Invest Med. (1993) 16:204–9.
143. Ford JT, Wong CW, Colditz IG. Effects of dietary protein types on immune responses and levels of infection with Eimeria vermiformis in mice. Immunol Cell Biol. (2001) 79:23–8. doi: 10.1046/j.1440-1711.2001.00788.x
144. Watanabe A, Okada K, Shimizu Y, Wakabayashi H, HiguchiK, Niiya K, et al. Nutritional intervention of chronic hepatitis by whey protein (non-heated). J Med. (2000) 31:283–302.
145. Ng T, Lam T, Au T, Ye X, Wan C. Inhibition of human immunodeficiency virus type 1 reverse transcriptase, protease andintegrase by bovine milk proteins. Life Sci. (2001) 69:2217–23. doi: 10.1016/S0024-3205(01)01311-X
146. Superti F, Ammendolia M, Valenti P, Seganti L. Antirotaviral activity of milk proteins: lactoferrin prevents rotavirus infection in the enterocyte-like cell line HT-29. Med Microbiol Immunol. (1997) 186:83–91. doi: 10.1007/s004300050049
147. Pourtois M, Binet C, Van Tieghem N, Courtois P, Vandenabbeele A, Thiry L. Inhibition of HIV infectivity by lactoperoxidase-produced hypothiocyanite. J Biol Buccale. (1990) 18:251–3.
148. Mikola H, Waris M, Tenovuo J. Inhibition of herpes simplex virus type 1, respiratory syncytial virus and echovirus type 11 by peroxidase-generated hypothiocyanite. Antiviral Res. (1995) 26:161–71. doi: 10.1016/0166-3542(94)00073-H